Building good posture with STEM
STEM Concept 1: Basic principles of physics
Mechanics is a branch of physics that studies the motion of objects and the forces that cause that motion. It is a fundamental area of study in physics and is divided into two main branches: classical mechanics and quantum mechanics.
Classical mechanics is the study of the motion of macroscopic objects, such as bicycles, cars, and airplanes, and the forces that cause that motion. Classical mechanics also includes the study of rigid body dynamics, which is the study of how rigid objects move and rotate. For instance, human bones are objects of a rigid body.
Quantum mechanics, on the other hand, is the study of the motion and behavior of particles on a microscopic level, such as atoms, electrons and photons. It includes the study of wave-particle duality, quantum states and superposition, and the uncertainty principle.
The basic theory of classical mechanics & physics is Newton's Laws. Newton's laws of motion are three physical laws that, together, laid the foundation for classical mechanics. They describe the relationship between a body and the forces acting upon it, and its motion in response to those forces. The laws are:
The first law, also known as the law of inertia, states that an object will remain at rest or in uniform motion in a straight line unless acted upon by an external force; this tendency to resist changes in a state of motion is inertia. This means that if there is no net force acting on an object, it will not accelerate. Inertia is the tendency of objects in motion to stay in motion, and objects at rest to stay at rest, unless a force causes its speed or direction to change.
The second law, also known as the law of acceleration, states that the acceleration of an object is directly proportional to the net force acting on the object, and inversely proportional to its mass. This means that the greater the force acting on an object, the greater its acceleration will be, and the greater the mass of an object, the smaller its acceleration will be. The equation for this is: F = ma, where F is the force acting on an object, m is the object's mass, and a is the object's acceleration.
The third law, also known as the law of action and reaction, states that for every action force, there is an equal and opposite reaction force. This means that when one object exerts a force on another object, the second object will exert an equal and opposite force on the first.
Newton's laws of motion are considered the foundation of classical mechanics, and are still widely used in physics today to describe the motion of objects and to solve problems involving forces and motion. They are also used in many other fields, such as engineering and astronautics, to understand and predict the behavior of physical systems.
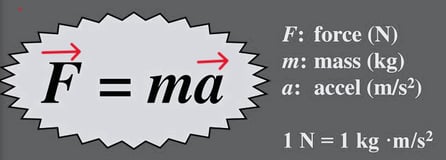
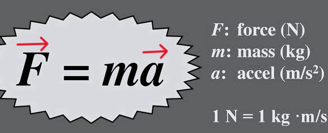
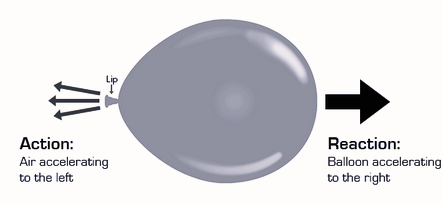
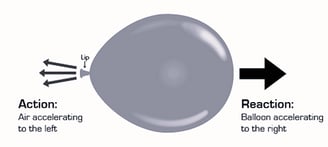
STEM Concept 2: Structural mechanics
Structural mechanics is a branch of mechanics that deals with the behavior of structures, such as buildings, bridges, and machines, under loads. It involves the study of how structures respond to the forces and loads that are applied to them, and how they deform and fail under those loads.
Structural mechanics is closely related to the field of solid mechanics, which is the study of the deformation and failure of solid materials under load. However, while solid mechanics deals with the behavior of individual materials, structural mechanics deals with the behavior of entire structures made up of many different materials. Structural mechanics includes the study of:
Statics: the study of how structures respond to loads that are in equilibrium, meaning that the net force and torque on the structure is zero.
Dynamics: the study of how structures respond to loads that are not in equilibrium, meaning that the net force and torque on the structure is non-zero.
Strength of materials: the study of how materials behave under loads, including how they deform and fail.
Stress and strain: the study of how loads are distributed within a structure and how they affect the internal state of the structure.
Deformation and stability: the study of how structures deform and how they maintain their stability under loads.
Structural mechanics is an interdisciplinary field that draws on the principles of physics, mathematics, and engineering. It plays an important role in the design, construction, and maintenance of many types of structures and is essential for ensuring the safety and durability of these structures
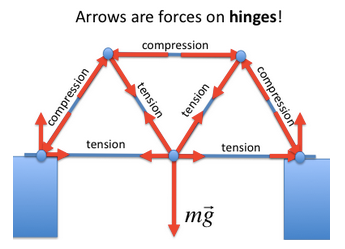
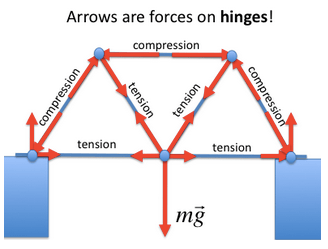
STEM Concept 3: Structural engineering
Structural engineering is a specialized field within civil engineering that deals with the design and analysis of structures such as buildings, bridges, towers, and other large-scale infrastructure. Structural engineers use their knowledge of mathematics, physics, and materials science to ensure that these structures are able to withstand the loads and forces that they will be subject to, both during construction and in their intended use.
Here are some specific areas of expertise within structural engineering:
Load analysis: determining the loads that a structure will be subjected to, such as the weight of the structure itself, the weight of the people and equipment that will occupy it, and environmental factors such as wind and earthquakes.
Material selection: selecting the appropriate materials for a structure, such as steel, concrete, wood, or composites, and determining the properties of those materials that are relevant to the design.
Structural analysis: using mathematical and computational methods to analyze the behavior of a structure under loads, including how it will deform and the internal stresses that will be present.
Design: using the information from the load analysis and structural analysis to create the plans and specifications for a structure, taking into account the necessary safety factors and building codes.
S tructural engineers work on a wide range of projects, from small-scale renovations to large-scale infrastructure projects. They they collaborate with architects, other engineers and construction professionals to ensure the safety and functionality of the structures. They also have to consider issues such as sustainability, durability, and aesthetics in their designs.
The basic concepts of structural engineering can be applied to body posture, such as the structure of sacrum and the pelvic.
T he sacrum and the pelvis form the pelvic girdle, which serves as the foundation for the transfer of loads from the upper body to the lower limbs and ultimately to the ground. The pelvic girdle acts as a stable base for movement and supports the weight of the upper body. The sacrum and the pelvic bones are connected by strong ligaments, providing stability and enabling the transfer of forces from the spine to the legs.
The pelvic girdle is composed of three bones on either side known as the ilium, ischium, and pubis which attach to the sacrum and femurs to connect the axial and appendicular skeletons. The pelvic girdle has several attachments, starting with the sacrum and ilium. The sacroiliac joint is held together by a combination of ligaments, including the sacroiliac ligaments, which attach the ilium to the sacrum. These ligaments play an important role in stability and support of the joint and the entire pelvic region.
Additionally, the sacrum and ilium have matching irregular contours that increase joint strength and stability. The pubic symphysis is a fibrocartilaginous joint located at the most ventral point where the two hemipelvises meet. The flexibility of the pubic symphysis allows for an increase in the function of the pelvis and enables movements such as walking and running.
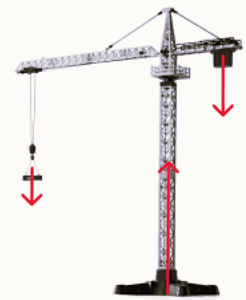
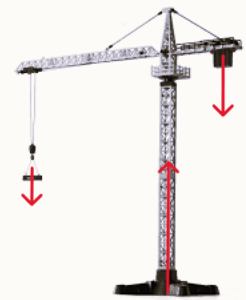
STEM Concept 4: Mechanostat Theory — Skeletal Adaptation
The mechanostat theory is a theory in the field of biomechanics that proposes that bone mass is regulated by the mechanical forces that are applied to it. The mechanostat theory offers a fascinating insight into how bones adapt to the stresses they encounter, a principle that’s incredibly relevant in physical training.
According to this theory, bone tissue isn't static; it's highly responsive to the mechanical forces exerted on it. When bones are subjected to stress, such as during weight-bearing activities, the cells responsible for bone remodeling—osteoblasts (which build bone) and osteoclasts (which break bone down)—are activated. This activity helps the bone adjust its mass and architecture to better handle the stress, essentially becoming stronger where it's needed most.
This adaptive mechanism ensures that bones are not only strong but also efficient. For instance, if a particular load or force is frequently applied to a bone, the bone remodels itself to handle that load more effectively by becoming denser. This is crucial not just for maintaining bone health but also for preventing fractures and other bone-related injuries.
This theory helps to explain why weight-bearing exercises and activities that involve loading the bones, such as resistance training and weightlifting, can help to promote bone health and prevent osteoporosis.
Body weight exhibits minimal day to day variation. However, static load and the resulting deformation of bone (mechanical strain) generated by weight depend upon posture. For example, standing on one leg places a higher load on weight bearing bones of the lower limb than standing on both legs, while transferring to a sitting or lying prone position alters the direction as well as reduces the magnitude of limb loading. These forms of skeletal loading are static. The mechanostat theory can extend to the Whole Skeleton Integration:
Extending the mechanostat theory, it’s evident that the entire skeleton, not just individual bones, adapts to dynamic actions. This systemic response is crucial for athletes who need precise coordination and force distribution across multiple bones and joints to perform complex movements effectively and safely.
The larger mechanical loads applied during physical activities such as walking subject loaded bones to higher peak strain levels. Skeletal loading associated with physical activity is dynamic because loads change rapidly in magnitude and direction and peak strain is maintained for short durations (ms). During walking, the lower limbs are subjected to peak loads equivalent to about 1.5 × body weight with the interval between strides being. More intense physical activities, such as those that occur in many sports, place even greater strains on loaded portions of the skeleton.
Implications for Athletes: For athletes, this theory underlines the importance of specific and repetitive training regimens. By repeatedly performing the same actions, athletes help their bones adapt to the specific demands of their sports. This repeated loading not only trains the muscles but also conditions the skeleton to manage and exert integrated forces across various bones, improving performance and precision. For example, a tennis player repeatedly practicing serves will develop stronger and more adapted arm and shoulder bones over time.
In essence, the mechanostat theory illuminates why targeted, repetitive training is so effective and necessary for athletes. It also emphasizes the importance of considering bone health as an integral part of overall fitness and athletic training, highlighting the need for activities that appropriately stress the bones to foster both strength and durability.
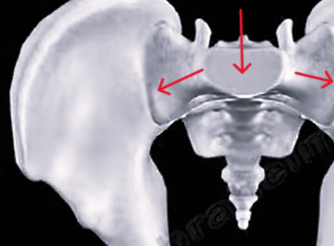
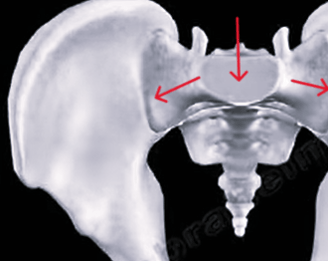